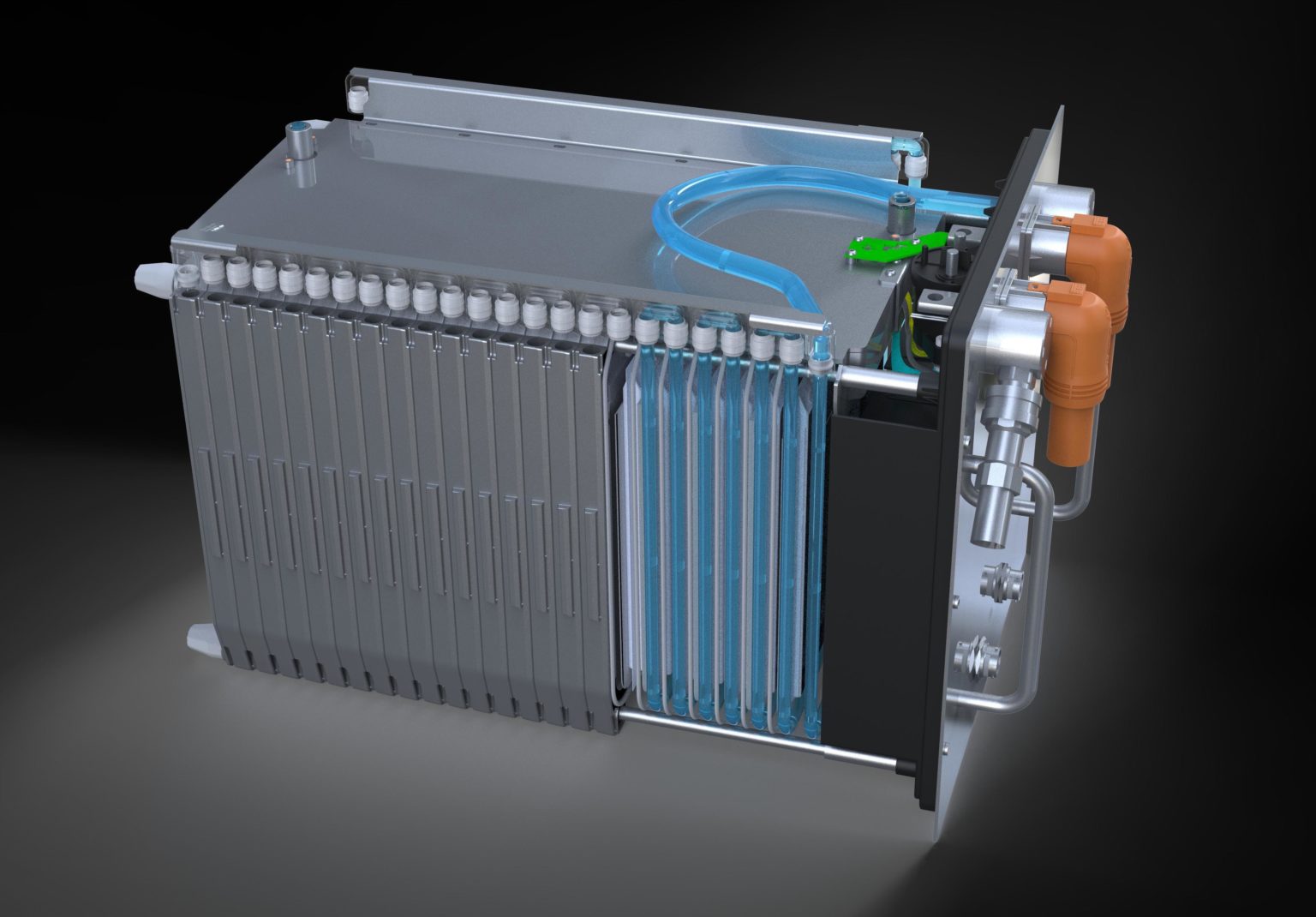
Energy storage safety for commercial vessels; lessons learned in practical application
Published in G Captain on February 3, 2020. https://gcaptain.com/advances-in-battery-safety-and-technology/
Forward
A Li-ion battery has several potential hazards that must be considered in order to keep people and equipment safe. It is important to consider these hazards for each stage of the battery life, not just the day it is installed. From manufacturing through to delivery, installation, commissioning, maintenance and eventually the recycling of the battery, safety must be first and foremost.
At the core of the system is the lithium-ion cell; a chemical power plant that cannot be simply switched off in order to make it safe. A battery cell is by its nature always live; therefore, it is important to ensure the safety of personnel from this potential hazard. The voltage on a single cell is low and therefore not hazardous, however the potential current is very high since the internal resistance of the cell is low. Shorting the positive and negative with a metal object will either result in the battery terminals evaporating or the metal object getting very hot.
During manufacturing, special tooling and processes are used to decrease the risk of short circuit of a cell. When the cells are assembled in series to form higher voltages collectively in a module, the voltage becomes more dangerous. Again, special assembly tooling is used to protect personnel against accidental shorting. Once assembled it is important to protect personnel from this voltage during transportation, installation, commissioning and de-commissioning.
For this, SPBES uses a contactor inside the module to isolate the battery voltage from the battery terminals. This contactor can only be closed by the Battery Management System (BMS) during operation, therefore eliminating all voltage related hazards when it is not in use. The total battery voltage once installed is very dangerous considering we commonly install batteries at bus voltages higher than 700VDC and up to 1500VDC. The protection for this is done through proper isolation design, the use of breakers and independent safety circuits like high voltage interlocks, independent breaker trips and ground fault detection.
The system is also designed to reduce the voltage anywhere on the installation to less than 100V when the system is off. This ensures that any unintentional shorts will not result in a dangerously high current and potential damage to the system.
Thermal runaway is a potentially catastrophic hazard that must also be in the front of an engineer’s mind when designing a lithium-ion battery. It is rare that thermal runaway occurs, but the impact can be devastating when it does . Our design philosophy is to eliminate the risk completely through our patented cooling system, CellCoolTM.
Rather than trying to manage the potential consequences of a thermal runaway event, SPBES has always focused on removing this hazard completely. Even if the cell heats up, regardless of the reason, SPBES’ system can remove the heat quick enough to prevent thermal runaway from occurring. Additionally, SPBES’s cooling system protects the cells from external heat sources such as a fire outside the battery.
Our philosophy has always been and always will be to protect the vessel and crew to the best of our ability, incorporating new safety innovations from our R&D team as they become available.

Advances in Battery Safety and Technology
Battery technology has evolved very quickly, but hybrid and electric marine propulsion is still a relatively young industry. As of today, there are still no commercial systems that can claim to have been in operation for 10 years. Despite this, the economic and environmental advantages of battery storage have meant that there are now dozens of ships operating in hybrid and full electric modes. By recent estimates, Energy Storage Systems (ESS) are now being incorporated in roughly 75% of refits and new build vessels around the world.
In 2009 I designed my first lithium batteries for marine applications. These were designed to demonstrate the principal that MW scale ESS could work like traditional propulsion while delivering real commercial value; and there were a lot of doubters. Today, we have evolved not only performance, but safety, integration, cost and risk management to much more predictable levels. The data obtained from constant commercial use of the battery system provided invaluable information that allowed us to evolve and continuously improve our systems.
The Classification Societies and Flag Authorities have also constantly pushed for better and better systems. These agencies have been incorporating powerful risk evaluation tools to ensure operator and passenger safety as system capacities got bigger and bigger.
Operational data and experience have led to significant improvements in battery design resulting in improved safety, system life, risk reduction and overall performance. The improved performance of modern marine batteries have also changed the market. Lower system cost means more and more marine verticals are now finding ROI from energy storage. For example, during the formation of the marine ESS industry, tugboats and ferries represented the best commercial applications for energy storage systems. Today we can add cruise ships, oil and gas, offshore and wind farms vessels to a never-growing list of commercial vessels that are making an ever-greater use of energy storage.
The key considerations of effective battery design start with two principal issues 1) an ESS must be an improvement to the existing methods of operating ships, and 2) the solution has to deliver financial benefits without external support (government grants or tax credit schemes) in order to permanently earn its place as a part of system design.
These are the key questions that we asked when at the design table for our current systems, understanding that they are the key to success. However, we also knew from years of real-world experience that the following criteria are also critically important to long term commercial success:
Safety: we had to be able to effectively eliminate the possibility of thermal runaway in a battery system, otherwise we will never see true acceptability in the markets and growth in system size. This challenge was at the top of our minds in every design decision, and we addressed it by creating our patented CellCoolTM cooling system that effectively removes the risk of thermal runaway.
The principal is very simple; reduce the temperature of the cells at a faster rate than the cell increases in temperature. No matter how hard you work them, with CellCoolTM they will not achieve the temperature required to go into thermal runaway. We worked in cooperation with Classification Societies and Flag Authorities to developed what we all felt were difficult to pass safety tests designed to demonstrate that batteries are inherently safe. We took this to another level and set our own gold standard as safety in the face of spontaneous combustion- the most difficult test that a battery can face.
Even in this very demanding test, we have proven success. Our systems are able to prevent significant damage to the battery (including propagation at the cell level) and ultimately make every system safe to operate. This is done with an inherently simple liquid cooling system. It cannot be achieved with air cooling systems due to the issue of managing transfer of heat with something as energy dense as most lithium chemistries.

Safety has other considerations as well; there is disaster safety at the cell level, and then there is safe use of batteries. We designed a BMS that is inherently focussed on protecting the ship, the battery system and the cells. This is done at its core by monitoring the voltage and temperature of every individual cell in the system, and then balancing the performance of the vessel within the safe operating principals of the ship.
There are two different ways to design a BMS; one that is ideal for a fully electric ship and one that suits hybrid applications. While both in principal will give the operators choices in the event of a battery failure, in a fully electric ship, the safe operation of the vessel becomes the guiding principal of the decision making of the BMS and Power Management System (PMS). In a hybrid application, the batteries can become the focus of the performance of the PMS as the vessel has alternative propulsion systems and is not totally reliant on the battery for operation. Once we define the type of application as either hybrid or electric, we can optimize the operational logic of the BMS as it pertains to the PMS/operator decision making criteria.
Another critical element of safety in design has been the inclusion of contactors in our building block modules. Basically, as we are building DC voltage systems that range from 300-1500VDC, the risk of personal injury in transportation and service are very high. For example, a 1500 VDC arc flash can permanently disable a technician. By adding contactors in the individual battery modules, we eliminate voltage at the terminals until the system is fully engaged and the BMS can approve that all cables are correctly sequenced and protected. There is no voltage or power on the terminals as long as they are open. We also reduce the risk by isolating the building blocks as single units no matter how large the overall size. The element of crew safety of our technicians and the operators of the vessels cannot be overstated in terms of benefit to our customers. We can now train ships engineers and crew to do maintenance on the batteries, we don’t need to bring in specially qualified electricians to do basic maintenance. This design decision was not free, but it is the right way to go to improve overall safety for our customers ships.

Cost: Another critical part of the design of a battery is not the actual battery itself, but the space the battery operates in. The impact of incremental costs of necessary added systems required for safe operations is significant. In most cases, and in our earlier designs, things like fire safety, fire detection, gas detection, gas extraction, cooling and emergency ventilation were left to other contractors and not included in the cost of the battery system. These so-called add-on systems are actually critical to the performance of a battery and are not optional, but in most cases battery suppliers will leave these added costs to the ships builders.
Our approach became more holistic and covered all parts of the battery system as our design evolved, which means less added cost per kWh and more integrated engineering. Our next evolution in development is to validate the design of our core modules to withstand an A60 battery test. Validation of this test will eliminate the need to build an A60 enclosure for the batteries.
To put this in context, one of our integrator partners determined that the typical added cost of a battery system could be as high as $275/kWh for a total battery installation, this cost comes on top of the cost of the batteries themselves.
It is essential that integrators and end customers always understand the overall system costs to allow decisions to be made on return on investment based on the actual installed system costs not just the battery cost. In fact, SPBES is not completely immune to this cost; our liquid cooling requires chillers sized to meet the power demand of the system and our gas extraction system must be correctly vented, but instead of $275/kWh, we face a cost typically of $20/kWh for the added components and to meet all performance requirements.
Another benefit of SPBES CellCool liquid cooling is the ability to actually predict the life of our systems. Air cooled systems are very dependent on the ambient battery room temperature to be able to manage the overall life of a lithium battery, and they are very fickle. Even a small increase in ambient battery room temperature will affect the temperature of the lithium cells andcan have a significant reduction in calendar life. In contrast, liquid cooling maintains the temperature of the cells at a fixed range and we can eliminate the impact of ambient temperature on the performance life of the cells.
As system life continues to be in the 10-year range and with many operators seeking longer-life solutions, eliminating temperature as a variable goes a long way to meeting lifespan requirements. There are still a large number of factors that will influence battery life, but temperature is by far the most impactful.

System Size: Another area where we have seen a significant evolution both in the use of cells and the design of a battery is size. Cell manufacturers have significantly improved energy density over the last ten years. By increasing the energy density of the lithium-ion cells, a significantly smaller system can be created. For example, a system with 88kWh per module versus a battery that has 65kWh per module has already achieved 35%improvement of weight and space required for an installation. As long as the cycle life meets the lifetime need, this is a huge improvement. In my experience, increases in energy density tend to a reduced cycle life.
The other significant feature of any system is the percentage of energy available on a continuous basis. On our first-generation air-cooled design, the continuous rating was about 70%. This meant that if we needed 1MW of energy, we needed to have about 1.4MWh of capacity to operate at 1MW load. This meant a larger, heavier system that was also significantly more costly to install and maintain. If we assumed that the battery system cost $100/kWh, then a 1.4MWh battery would add $140,000 to the capital cost of the system – and does not even consider the ongoing performance and financial impacts of the increased size, weight and maintenance!
A liquid cooling system allows SPBES to use significantly more of the battery capacity. This really means we can greatly reduce the size and the associated costs of the battery. In our case, the battery can now operate at an average continuous rate (charge and discharge) of 300%. In the example above, a 1MWh system can now be met with a 350kWh battery; much smaller, much lighter, and much less costly to install, with only a $35,000 budget needed (if the necessary costs were $100/kWh).

While the above model is not always accurate, it is more reflective in what we call power systems. In energy systems where a slower, more steady output of energy is required, size is totally dependent on capacity. Clients and operators will understand the concept that their vessels are operating underpower or energy requirements and where energy is the driver, we have engineered a battery system where we can simply use the same battery with all of the same components, but with cells that have higher energy density- so we can significantly reduce the footprint of the battery system.
Sustainability: A battery that can last for ten years is a pretty amazing thing, but it will never match the lifespan of the vessel itself. This equates to significant costs to replace a battery system every five or ten years on a vessel that will be in service for up to 50 years. We took this challenge, and in analyzing a system, realized that while all components will require some maintenance, the most significant reason for ESS replacement was the fact the cells will age.
The answer to continuous replacement (and the capital costs associated with it) is a design that allows us to remove and renew the cells regularly, i.e. every 5 to 10 years. If we can keep the bulk of the infrastructure and safety systems in place, then we can reduce the cost of replacing the system to the cost of cell replacement and cell recycling. This radically reduces the total cost of operating an electric or hybrid vessel over its lifetime.
Cell SwapTM has been at the core of our design since 2015, with every module core (regardless of chemistry of the cells) able to be replaced within 30 minutes. SPBES technicians can perform CellSwap refurbishment while the ship is running or in for maintenance. Cell swap means that the battery system life span is now the same as that of the vessel; it is similar to engine maintenance and rebuilds. With this inclusion, the design of the battery system is now in line with marine market requirements.
Recycling will have an increasingly prominent role in decision making over the next generation. This is part of the benefit of a cell swap; we can safely support the recycling of the lithium cells and do this at a very low cost and impact of operational budgets. Part of our contracting now is to include end of life recycling with every system. While often overlooked, it is necessary for any company that uses ESS in commercial operations to include this operational expenditure in their impact analysis of utilizing energy storage systems in day to day application.
Where to next? I think that commercial needs are going to continue to drive improvements. Natural evolution of clean maritime propulsion with products such as fuel cells will significantly improve environmental impact and cost-effective operations. Organizations like the IMO and Class and Flag Authorities will continue to motivate us to move the technology forward in order to meet the needs not only of our industry, but those of society as well. We look forward to the challenges of the future and the next generation of developments to come.